Unveiling the Brain’s Excitatory Superhighway in Glutamatergic Projections
Glutamatergic projections are the primary source of excitatory neurotransmission in the central nervous system (CNS) influencing many brain functions from learning and memory to motor control and sensory processing. Understanding the role of glutamate and the neural networks it influences is key to understanding both normal brain function and the pathophysiology of many neurological and psychiatric disorders.
The Role of Glutamate: An Overview of Function and Significance
Glutamate is not only the most abundant neurotransmitter in the brain but also a key player in many metabolic processes. Glutamate is packaged into small containers or ‘vesicles’ within neurons. When a signal arrives, glutamate is released into the gap between neurons (the synapse) where it binds to specific receptors on the next neuron, to send the signal along, known as neurotransmission.

However, glutamate plays more of a role than excitation. We now know that glutamate is critical for synaptic plasticity, the ability of synapses to strengthen or weaken over time in response to changes in activity. This plasticity is fundamental to learning and memory, and adapting to new information. Glutamate is also involved in neuronal development, regulating important cellular processes including migration, differentiation and synapse formation. Disruptions in glutamatergic signalling (such as increased or decreased release) is linked to the pathophysiology of many neurological conditions, and highlights the importance of glutamate for healthy brain function.
The Link Between Glutamatergic Imbalance and Neurological Disorders
Glutamatergic dysregulation is involved in many neurological and psychiatric conditions including:
- Schizophrenia: Glutamatergic abnormalities, particularly linked to hypoactive NMDA receptor signalling, have been shown to contribute to the pathophysiology of schizophrenia. Some antipsychotics modulate glutamatergic activity.
- depression,
- anxiety,
- addiction,
- ptsd.
Mapping the Major Glutamatergic Projections in the Brain
Glutamatergic neurons are spread throughout the brain and form specific projection pathways that connect different brain regions. Some of the main glutamatergic projections are:
- Cortico-cortical: These connect different areas within the cortex, allowing communication between sensory, motor and association areas. Crucial for higher level cognitive functions like decision making, language and spatial reasoning.
- Cortico-thalamic: The cortex sends glutamatergic projections to the thalamus, a key relay station for sensory info. These modulate thalamic activity and influence the flow of info to the cortex.
- Thalamo-cortical: The thalamus sends glutamatergic projections back to the cortex, the primary excitatory input to cortical neurons. This back and forth communication is essential for cortical excitability and processing sensory info.
- Cortico-striatal: The cortex goes to the striatum, a big part of the basal ganglia that helps with motor control, habit formation and reward learning. Glutamate from the cortex feeds into striatal activity and controls these functions.
- Hippocampal: The hippocampus, the learning and memory part of the brain, gets glutamate from the entorhinal cortex and sends glutamate to other parts of the brain including the cortex and amygdala. These projections are for encoding and retrieving memories.
Glutamatergic transmission is the behind the scenes worker of learning and memory, LTP is its superpower. LTP is a long term strengthening of synapses based on recent activity patterns, necessary for encoding memories. This is most evident in the hippocampus, a part of the brain involved in memory formation. The process of LTP is complex, involves NMDA receptors activation which requires both glutamate binding and postsynaptic depolarization to be activated. This “coincidence detection” mechanism ensures that synapses are strengthened only when both pre and post neurons are active at the same time, according to Hebbian learning : “neurons that fire together, wire together”.

LTP involves several steps: glutamate binding to NMDA receptors, calcium influx triggering intracellular signaling pathways and insertion of more AMPA receptors into the postsynaptic membrane, increasing synaptic strength. LTP has three main properties: cooperativity, multiple synapses need to be activated; input specificity, strengthening is specific to activated synapses; associativity, weak stimuli can induce LTP when paired with strong stimuli. Neurotrophic factors like BDNF play a critical role in LTP and learning, increasing synaptic plasticity and memory consolidation. Understanding LTP is key to understanding neurological disorders, like Alzheimer’s disease and addiction, where manipulating NMDA receptors can have a big impact on memory. By unlocking the secrets of glutamatergic projections and LTP researchers can develop new therapeutic strategies for memory related disorders and hope for better cognitive functions in the future.
Modulation of Glutamatergic Transmission: Receptors, Transporters, and Glial Interactions
Glutamatergic transmission is a key part of the central nervous system (CNS), involved in learning, memory and synaptic plasticity. This transmission is modulated by many factors, including receptors, transporters and glial interactions. Understanding these components is important for neuropsychiatric conditions where glutamate is dysregulated.
Receptors
Glutamate receptors are divided into two main categories: ionotropic and metabotropic.
- Ionotropic Glutamate Receptors (iGluRs): These include AMPA, NMDA and kainate receptors. They are ligand gated ion channels that facilitate fast synaptic transmission by allowing ions to flow into the neuron, leading to depolarization and neuronal excitation.
- Metabotropic Glutamate Receptors (mGluRs): These receptors are G-protein coupled and modulate synaptic transmission through intracellular signalling pathways. They can affect the activity of voltage gated ion channels and regulate neurotransmitter release.
Transporters
Glutamate transporters are essential for keeping glutamate in balance in the synaptic cleft. These transporters, mostly located in glial cells, uptake glutamate to prevent excitotoxicity and ensure proper synaptic function. pH can modulate the activity of these transporters, affecting synaptic transmission.
Glial Interactions
Glial cells, especially astrocytes, play a significant role in modulating glutamatergic transmission. They regulate glutamate uptake and release, influencing synaptic plasticity and neuronal excitability. Glial dysfunction can lead to glutamate dysregulation, contributing to many neuropsychiatric conditions.
Clinical Implications
Glutamatergic dysregulation is involved in many neuropsychiatric disorders:
- Depression: Glutamatergic abnormalities are associated with depression, with ketamine targeting glutamate to improve treatment outcomes.
- Anxiety and Schizophrenia: Glutamate dysregulation is also involved in these conditions, so potential therapeutic targets.
- PTSD and OCD: Hyperarousal and intrusive memories in PTSD, and compulsive behaviours in OCD, may be related to glutamate dysregulation.
- Neuroinflammation: This can lead to glutamate dysregulation, contributing to depression and schizophrenia.
Future Directions
- Gene Therapies: Targeting genetic mutations that affect glutamate systems could be a new therapeutic avenue.* Biomarkers: Glutamate biomarkers for diagnosis.
- Diet: Ultra processed foods and MSG and glutamate levels and neuropsychiatric health. Get to the heart of glutamate.
Advanced Neuroimaging Techniques for Studying Glutamatergic Projections In Vivo

With advances in neuroimaging we have some great tools to study glutamatergic projections in the living brain. Here are a few:
- Magnetic Resonance Spectroscopy (MRS): MRS is a noninvasive way of measuring glutamate levels in specific brain regions, providing insights into local glutamatergic metabolism, which can be used as a proxy for underlying neural activity.
- Positron Emission Tomography (PET): PET can be used to image certain glutamate receptors and (to a lesser extent) transporters, allowing researchers to map the location of these proteins in the brain. Radioligands targeting specific glutamate receptor subtypes are under development, and may one day enable us to visualise glutamatergic transmission more precisely.
- Functional Magnetic Resonance Imaging (fMRI): fMRI doesn’t directly measure glutamate activity, but it can detect the functional consequences of glutamatergic transmission. By measuring the changes in blood oxygenation during cognitive tasks that rely on glutamatergic signalling, fMRI can help researchers to observe patterns of brain activity that are involved in glutamate related processes.
Current Research and Therapeutic Targets in Glutamatergic Neurotransmission
Current research is focused on developing new therapeutic strategies that target glutamatergic transmission for the treatment of neurological and psychiatric disorders. Some areas to consider:
- Glutamate receptors: Developing drugs that selectively target different glutamate receptor subtypes could lead to targeted treatments for specific disorders. For example drugs that increase NMDA receptor function may improve cognitive function in Alzheimer’s, while drugs that block AMPA receptors may reduce seizure activity in epilepsy.
- Glutamate transporters: Increasing glutamate transporter activity could reduce excitotoxicity and protect neurons in stroke and other neurological conditions.
- Glial-neuronal interactions: Modulating glial-neuronal interactions could be a new way to regulate glutamatergic transmission. For example drugs that stimulate astrocyte glutamate uptake could help restore glutamate homeostasis in disorders such as schizophrenia.
Highlands Recovery, located near Sydney, Australia, uses behavioural medicine to support clients through their intensive residential programs. These programs address trauma and related disorders without medication, using biological, psychological and social tools to stabilise and recover. Our goal is to empower individuals to achieve long term wellness through individualised care.
Reviewed by: Dr. Emma Bardsley
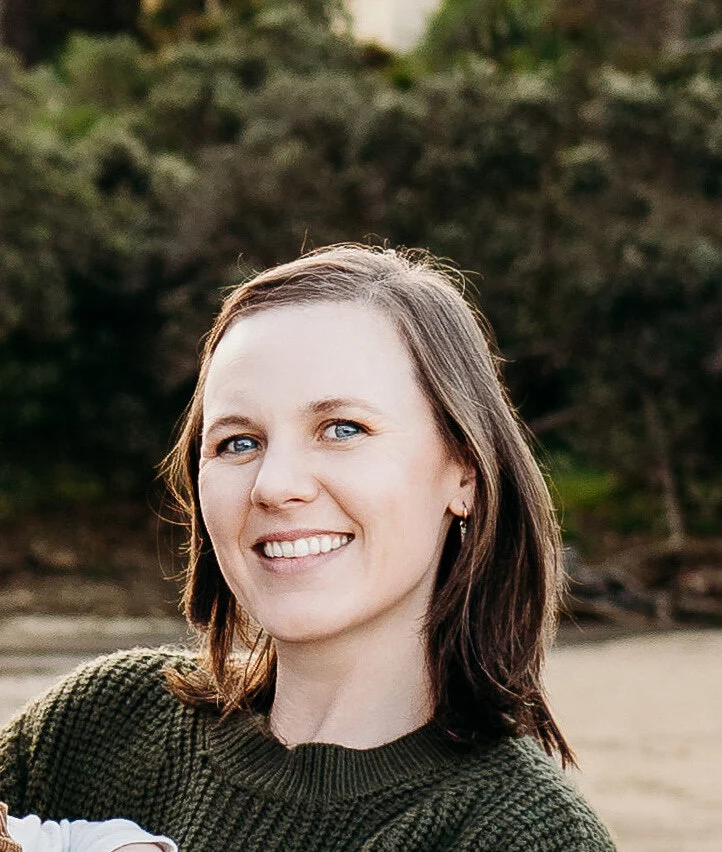
Dr Emma Bardsley is a neuroscientist with a PhD from Oxford and a post doctorate from Auckland University, along with an undergraduate degree in Pharmacology from King’s College London. She has lectured extensively on neuroscience, physiology, and pharmacological interventions, bridging foundational research and its clinical applications. With a strong record of publications in high-impact journals and extensive experience in scientific writing, editing, and peer review, she excels at translating complex research into practical insights. Based in New Zealand and collaborating internationally, Emma is dedicated to advancing understanding and treatment in the fields of trauma, addiction, and recovery.
References
Nature. (2002). Neural mechanisms of cognitive flexibility. Retrieved from https://www.nature.com/articles/nature00790
PubMed. (1991). Neurobiological substrates of psychiatric disorders. Retrieved from https://pubmed.ncbi.nlm.nih.gov/2072608/
PubMed. (1995). The role of neurotransmitters in mood regulation. Retrieved from https://pubmed.ncbi.nlm.nih.gov/7669016/
American Journal of Psychiatry. (2001). Long-term effects of childhood trauma. Retrieved from https://psychiatryonline.org/doi/full/10.1176/appi.ajp.158.11.1818
Nature Reviews Neuroscience. (2002). The neural basis of habit formation. Retrieved from https://www.nature.com/articles/nrn701
PubMed. (2006). The impact of stress on neuroplasticity. Retrieved from https://pubmed.ncbi.nlm.nih.gov/16894061/