Dopamine: The Neurotransmitter at the Heart of Reward and Motivation
Dopamine is a major neurotransmitter that controls movement, motivation, reward processing and many other physiological and psychological functions. As a member of the catecholamine family dopamine is made in the brain and peripheral tissues through a specific enzymatic pathway. It influences many neural pathways and behaviours, emotions and even responses to external stimuli. This article looks at the biochemistry of dopamine, its synthesis, receptor interactions and its importance for neurological health and function.

Dopamine is a catecholamine neurotransmitter synthesised in a two step enzymatic process from the amino acid L-tyrosine. Firstly, tyrosine is converted to L-DOPA by tyrosine hydroxylase and then decarboxylated to dopamine by aromatic L-amino acid decarboxylase (also known as dopa decarboxylase) . Dopamine acts through 5 receptor subtypes (D1-D5) which are G-protein coupled receptors (GPCRs) divided into D1-like (D1, D5) and D2-like (D2-D4) families. D1-like receptors activate adenylate cyclase via Gs proteins, increasing cAMP production, while D2-like receptors inhibit cAMP through Gi/o protein coupling.
Voluntary movement is controlled through activation of the nigrostriatal pathway, originating in the substantia nigra pars compacta and projecting the dorsal striatum. Degeneration in this region is thought to underpin the movement disorder and tremor seen in Parkinson’s disease. The mesolimbic pathway that includes projections from the ventral tegmental area (VTA) to the nucleus accumbens (NAc) is known to be involved in reward seeking and goal-oriented behaviours. Dopamine release in the NAc reinforces actions linked to positive outcomes, a process hijacked in addiction. Recent research also shows dopamine’s role in the mesocortical pathway which modulates executive functions via prefrontal cortex (PFC) connections. This pathway is for decision making and planning and dopamine balances exploration and exploitation in novel situations.
The Role of Dopamine in Neurological Disorders: A Clinical Perspective
Dopamine dysfunction is at the core of Parkinson’s, schizophrenia and restless legs syndrome. Levodopa (L-DOPA), a dopamine precursor, is also administered therapeutically as a gold-standard treatment but induces dyskinesias due to pulsatile receptor stimulation. New approaches include continuous intrajejunal levodopa-carbidopa gel infusion (Duodopa) and subcutaneous foslevodopa/foscarbidopa pumps, both designed to provide continuous dopaminergic stimulation, reducing motor fluctuations and dyskinesia in Parkinson’s patients. These aim to improve quality of life by reducing “off” times and increasing motor stability.
Dopamine Related Neurological Conditions:
- Parkinson’s Disease: Tremors, muscle stiffness, motor control issues due to loss of substantia nigra dopaminergic neurons. Levodopa and continuous dopaminergic stimulation (Duodopa) are treatments.
- Schizophrenia: Hyperactive mesolimbic D2 receptors causing positive symptoms (hallucinations) and reduced prefrontal cortex dopamine leading to cognitive deficits. Second gen antipsychotics like aripiprazole balance dopamine.
- Restless Legs Syndrome: Dopamine dysfunction, treated with dopaminergic drugs.
- Attention Deficit Hyperactivity Disorder (ADHD): Reduced dopamine signaling, focus and impulse control issues. Methylphenidate increases dopamine.
- Depression: Low dopamine levels contribute to mood changes, cognitive changes, sleep issues.
- Addiction: Dopamine dysregulation in reward pathways causes compulsive behaviors and substance dependence.
In schizophrenia, hyperactivity of mesolimbic D2 receptors is implicated in positive symptoms (e.g. hallucinations) while decreased dopamine bioavailability in the PFC contributes to cognitive deficits. Second-generation antipsychotics like aripiprazole act as partial D2 agonists to balance these pathways.
Dopamine and Addiction: Understanding the Neurobiological Mechanisms
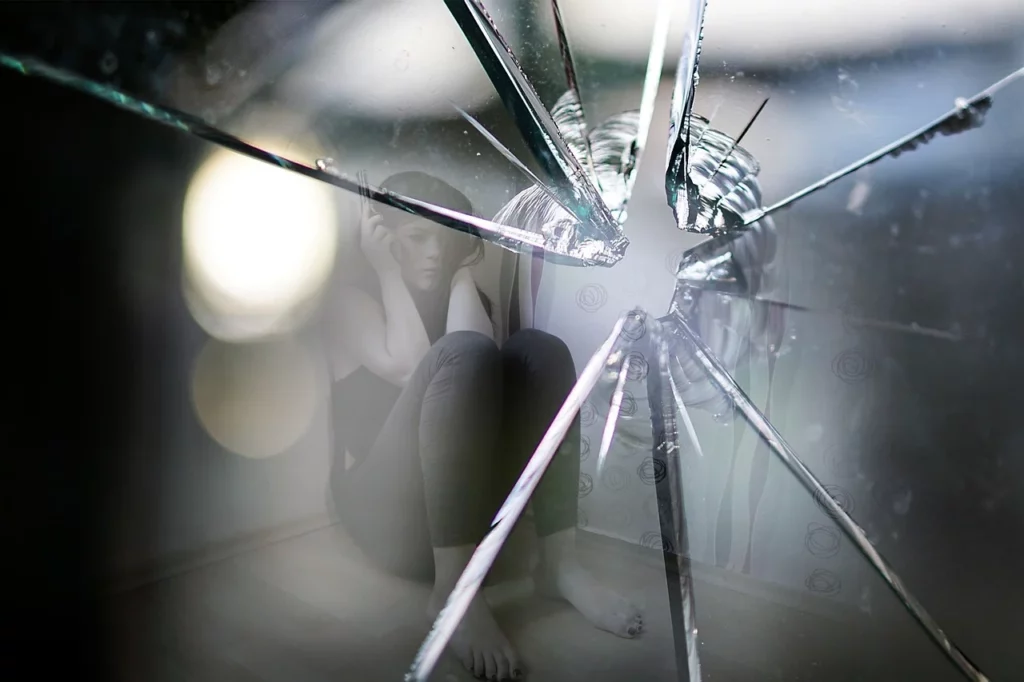
Addiction comes from maladaptive flexibility in the mesolimbic pathway. Drugs like cocaine and amphetamines increase synaptic dopamine by blocking the dopamine transporter (DAT) or promoting vesicular release. Chronic substance use leads to dopamine D2 receptor downregulation in the striatum, reducing sensitivity to natural rewards and reinforcing drug seeking behaviour
Dopamine also interacts with glutamate in the NAc to strengthen synapses through long-term potentiation (LTP), embedding drug associated memories. NMDA receptor antagonists like ketamine show promise in disrupting these memories, reducing relapse. Furthermore, research on dopamine-glutamate interactions highlights the role of synaptic plasticity in addiction, where drug exposure changes the strength and connectivity of neural circuits. This informs new therapeutic approaches.
The Impact of Dopamine on Cognitive Functions: Memory, Attention, and Learning
Dopamine’s role in cognition is evident by its regulation of the prefrontal cortex (PFC) where dopamineD1 receptors enhance working memory and attentional control However, dopamine appears to follow an inverted-U response, where both high and low levels of dopamine impair cognitive performance. Emerging research suggests that dopamine also mediates reward prediction error (RPE), which describes the difference in reward between expected and actual outcomes. Data from intracranial recordings have demonstrated that dopamine neurons in the VTA are activated during social interactions, driving adaptive learning. This is the mechanism underlying reinforcement learning where positive outcomes strengthen behavior. Recent advances in neuroimaging allowed researchers to map these processes in real-time, to see how dopamine modulates cognitive flexibility and decision making.
Dopamine’s Involvement in Mood Regulation: Implications for Depression and Anxiety
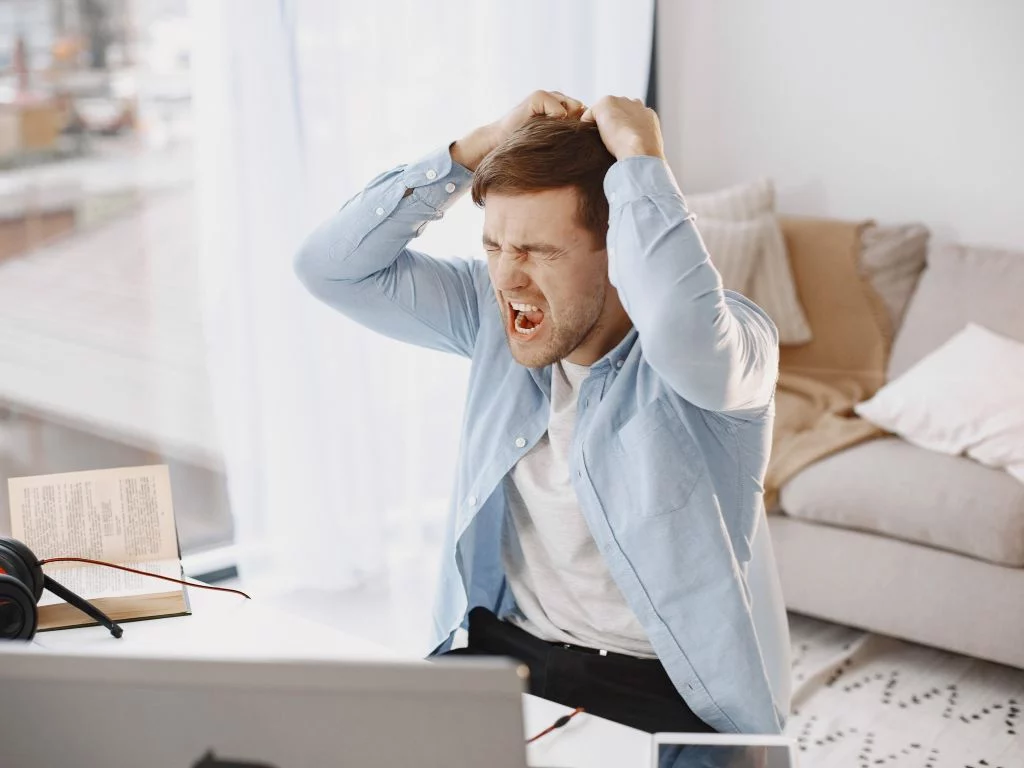
While serotonin is commonly associated with depression, dopamine also plays an important role in mood regulation. Anhedonia, a core symptom of depression, is predominantly linked to reduced dopamine transmission in the ventral striatum and PFC. Dopamine transporter (DAT) inhibitors such as bupropion, increase synaptic dopamine by preventing it’s reuptake into synaptic terminals. Excessive dopamine D2 receptor activity in the amygdala amplifies anxiety by increasing threat perception.
New therapies are targeting trace amine-associated receptor 1 (TAAR1) which modulates dopamine and serotonin release. Research into dopamine’s interaction with other neurotransmitters like GABA and acetylcholine is showing the complex interplay in mood regulation and potential new targets.
Dopamine Receptors and Their Role in Modulating Behavioural Responses
D1 and D2 receptors have opposing effects on cyclic AMP (cAMP) that results in alternative approach-avoidance behavioural responses.This receptor diversity allows dopamine to regulate a wide range of physiological and psychological processes.
More recent studies have also looked at the role of dopamine receptor heteromers in modulating synaptic plasticity and behaviour. These heteromers can change the pharmacological profile of drugs targeting dopamine receptors and open up new avenues for therapy. Understanding these interactions is key to designing better drugs with fewer side effects.
Dopamine Signalling Pathways: Recent Advances in Neuroimaging and Research
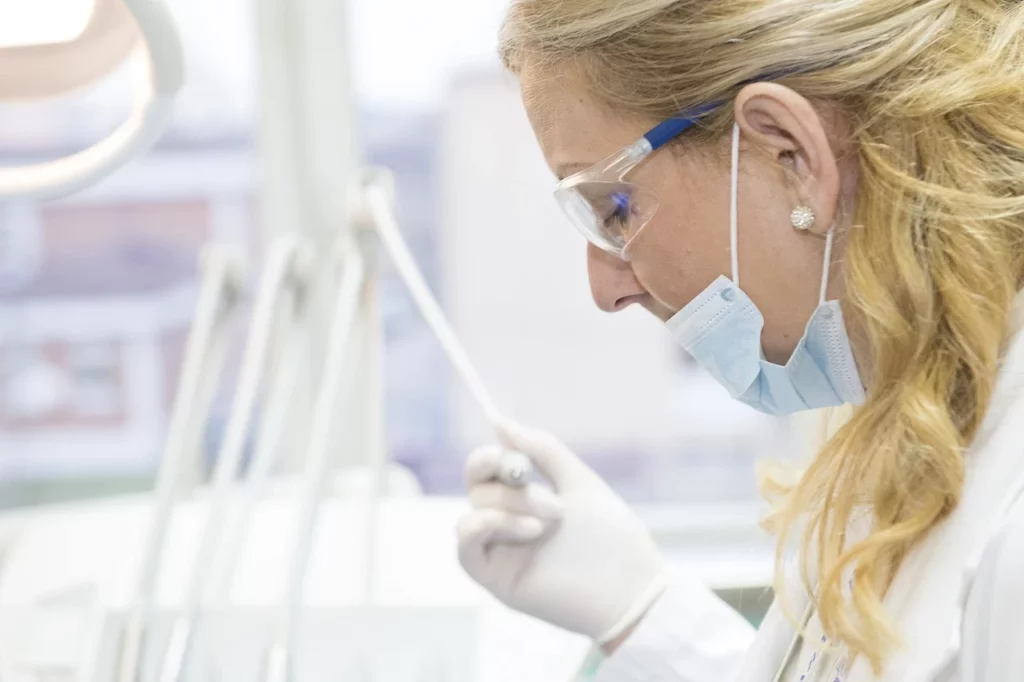
7T MRI is a big step forward in neuroimaging, with unprecedented spatial resolution to visualize dopaminergic nuclei like the substantia nigra. This clarity is critical for early detection of Parkinson’s disease as it allows you to see subtle changes in the structure of the neurons before motor symptoms appear. The higher signal to noise and contrast to noise ratios of 7T MRI also allows us to see microstructural changes related to various neurological conditions like iron deposition and vascular abnormalities in Parkinson’s and multiple sclerosis. Clinically it allows us to precisely place deep brain stimulators for Parkinson’s treatment and evaluate dopamine related tumor features more sensitively.
7T MRI can now see dopaminergic nuclei like the substantia nigra like never before, helping with early Parkinson’s diagnosis. The PET ligand [11C]-(+)-PHNO is a great tool for studying dopamine pathways, especially through its binding to the high affinity state of D2 receptors (D2-high) and selectivity for D3 receptors.
PET ligands like [11C]PHNO bind to D3 receptors and show receptor availability in addiction. Optogenetics shows that VTA dopamine neurons fire in sync with hippocampal theta waves during memory consolidation, demonstrating cross-regional connectivity. These tools have changed our understanding of dopamine’s role in cognition and disease and allow us to intervene precisely.
Plus, fast-scan cyclic voltammetry allows us to measure dopamine in real-time, and we’ve used this to study dopamine’s role in decision-making. We’ve shown that dopamine encodes value updates relative to past experiences in social interactions and learning. This is dopamine’s role in adaptive learning and social cognition.
Therapeutic Interventions Targeting Dopamine Dysregulation: Current Developments and Future Directions
AXO-Lenti-PD is a gene therapy that treats the root cause of dopamine deficiency in Parkinson’s disease by delivering three key enzymes for endogenous dopamine production into the brain. It’s administered through a single lentiviral vector and encodes TH, CH1 and AADC—the enzymes that convert L-tyrosine to dopamine
AXO-Lenti-PD delivers tyrosine hydroxylase genes via lentiviral vectors to restore dopamine synthesis. D3 receptor antagonists (e.g. VK4-116) reduce cocaine-seeking in preclinical models by normalizing NAc hyperactivity. Personalized levodopa dosing guided by COMT genotype minimises dyskinesias: Met/Met homozygotes need lower doses because they metabolise dopamine slower. COMT is the enzyme that degrades catecholamines, including dopamine, epinephrine and norepinephrine. It’s particularly important in the prefrontal cortex (PFC) where dopamine transporter (DAT) is low. COMT adds a methyl group to catecholamines via S-adenosyl methionine (SAM) and inactivates them. This is important for dopamine regulation and brain neurotransmission
These tailored approaches are the new face of precision medicine, using genetic and imaging data to optimize treatment.
Modern approaches to dopamine-related disorders are all about precision medicine, using genetic and imaging data to tailor treatments. Facilities like Highlands Recovery fit into this model, using evidence-based approaches that integrate neurochemical insights into structured support systems. Their programs are scientifically rigorous because we now understand dopamine’s many roles. By combining latest research with comprehensive care models, these centers can improve outcomes and quality of life for those with dopamine dysregulation.
Reviewed by: Dr. Emma Bardsley
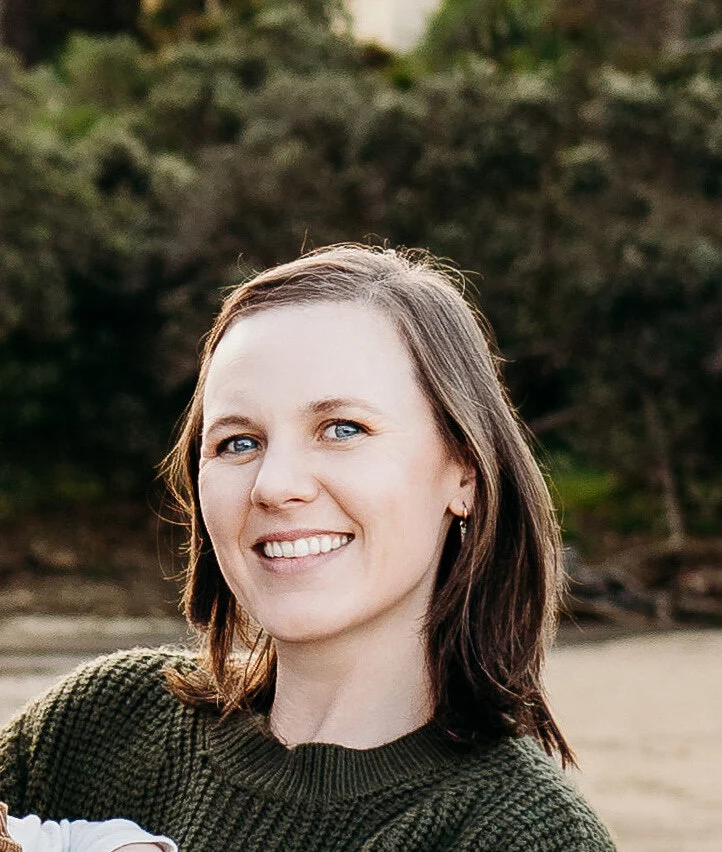
Dr Emma Bardsley is a neuroscientist with a PhD from Oxford and a post doctorate from Auckland University, along with an undergraduate degree in Pharmacology from King’s College London. She has lectured extensively on neuroscience, physiology, and pharmacological interventions, bridging foundational research and its clinical applications. With a strong record of publications in high-impact journals and extensive experience in scientific writing, editing, and peer review, she excels at translating complex research into practical insights. Based in New Zealand and collaborating internationally, Emma is dedicated to advancing understanding and treatment in the fields of trauma, addiction, and recovery.
References
Hyman, S. E., Malenka, R. C. & Nestler, E. J. Neural Mechanisms of Addiction: The Role of Reward-Related Learning and Memory. Annu. Rev. Neurosci. 29, 565–598 (2006). https://doi.org/10.1146/annurev.neuro.29.051605.113009
Bromberg-Martin, E. S., Matsumoto, M. & Hikosaka, O. Dopamine in Motivational Control: Rewarding, Aversive, and Alerting. Neuron 68, 815–834 (2010). https://doi.org/10.1016/j.neuron.2010.11.022
Koob, G. F. & Volkow, N. D. Neurobiology of addiction: a neurocircuitry analysis. Lancet Psychiatry 3, 760–773 (2016). https://doi.org/10.1016/s2215-0366(16)00104-8
Lupien, S. J., McEwen, B. S., Gunnar, M. R. & Heim, C. Effects of stress throughout the lifespan on the brain, behaviour and cognition. Nat. Rev. Neurosci. 10, 434–445 (2009). https://doi.org/10.1038/nrn2639
Campo, N. del, Chamberlain, S. R., Sahakian, B. J. & Robbins, T. W. The Roles of Dopamine and Noradrenaline in the Pathophysiology and Treatment of Attention-Deficit/Hyperactivity Disorder. Biol. Psychiatry 69, e145–e157 (2011). https://doi.org/10.1016/j.biopsych.2011.02.036
Petzinger, G. M. et al. The Effects of Exercise on Dopamine Neurotransmission in Parkinson’s Disease: Targeting Neuroplasticity to Modulate Basal Ganglia Circuitry. Brain Plast. 1, 29–39 (2015). https://doi.org/10.3233/BPL-150021